Vanillin – the compound responsible for the aroma and taste of vanilla (in our ice cream, chocolates and so on) – can now be produced from waste plastic bottles. Yes, you heard it right. Researchers from the University of Edinburgh, Dr. Stephen Wallace (Senior Lecturer at Institute for Quantitative Biology, Biochemistry and Biotechnology (IQB3) and also a UKRI Future Leaders Fellow) and Dr. Joanna Sadler (Post-doctoral Fellow in Wallace Lab) have found a way to directly upcycle poly(ethylene terephthalate) (PET) plastic bottle into vanillin – via enzymatic biotransformation. The work is published in the journal ‘Green Chemistry’ as Open Access [1].
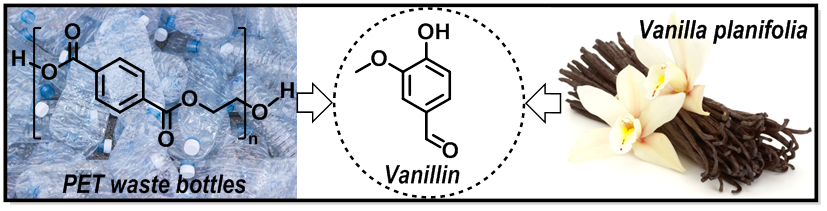
PET has been widely used in textiles and packaging applications, and manufactured 70 million tonnes annually [2]. However, most of them ends up in landfill, oceans or littering in the cities after a single-use application. From a ‘Circular Economy’ perspective, most of the research has been focused on recycling of PET into its starting monomers, terephthalic acid and ethylene glycol or bis(2-hydroxyethyl) terephthalate to ‘Close-the-Loop’ and produce pristine quality PET [3,4]. Wallace and Sadler instead focused on upcycling it into a chemical that already has a high market value. Their strategy contributes to the ‘Open-Loop-Recycling’ of Circular Economy [5] – meaning “upcycling of a material into a high or similar value material after its end-of-life” – by fully utilizing the inherent value embedded in it – so that it does not enter into the waste stream and stays in the economy!
Vanillin is a naturally occurring phenol (3-methoxy-4-hydroxy benzaldehyde) and is extracted from the cured seed pods of the orchid ‘vanilla planifolia’– which has an expected market value of USD 734.5 million in 2025 (CAGR of 7.9% from 2019), accounting to its uses as flavouring agent in food and beverage industry, fragrances in cosmetic and detergent industry, and pharmaceuticals [1,6,7]. It is also a value-added chemical for the production of a wide range of thermoplastic and thermosetting polymers. Vanillin has a very high demand (exceeded 37 000 tonnes in 2018) which cannot meet solely by its natural supply. 1 kg of vanillin production requires 500 kg cured vanilla pods – meaning an approximate of 40,000 pollinated flowers, and the whole process takes more than a year. Thus, vanillin is also produced industrially – approximately 85% of market is based on petro-based route such as reaction of guaiacol with glyoxylic acid or formaldehyde and rest 15% is produced via oxidation of lignosulfonates, a byproduct of wood-pulping industry. These comes under the ‘synthetic vanillin’ grade. Growing environmental concerns and the need for a ‘natural grade’ vanillin directed towards its synthesis via biotechnological conversion using microorganisms such as fungi or bacteria. In this regard, fermentation of vanillin using ferulic acid as substrate has been successful industrially. Other substrates such as glucose, glycerol, xylose, L-tyrosine, eugenol, isoeugenol, curcumin and so on, were also explored.
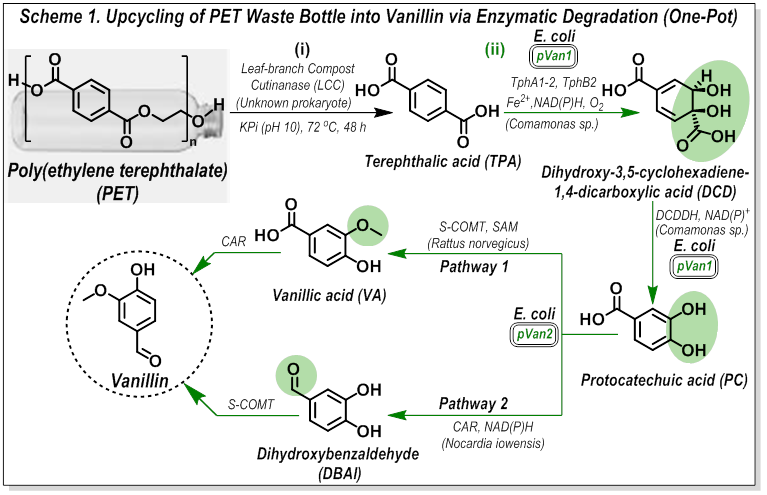