"Yesterday I was someone’s face mask, today I got upgraded into high value carbon fibers”.
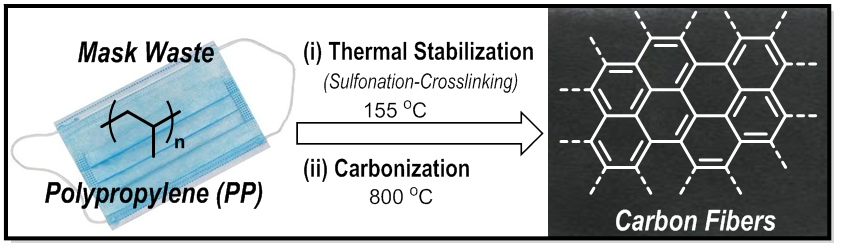
The current plastic pollution is worsened by COVID-19 pandemic, it was estimated that ~3.4 billion single use face masks were disposed of daily worldwide in mid 2020. The mismanaged waste was found littering the streets/roads and beaches, which not only has adverse affect on human health but it is also a threat to marine life. These single use/surgical face masks were made from nonwoven polypropylene (PP) fibers and are not biodegradable, thus can persist in the open environment and in the oceans for many years. Over time, they can disintegrate to form microplastics which can be swallowed by marine organisms – ultimately negatively impacting human health through food consumption. Most of the collected waste is landfilled – which also imposes pressure on land due to the staggering amount of waste, and most importantly the economic value invested for its production is also lost (~ 1.6 million tons per day produced in 2020). The incineration of these mask waste can recover some value in the form of energy, however it contributes to greenhouse gas emissions [1-3]. Urgent solutions are needed to address these issues. Researchers from School of Polymer Science and Engineering, The University of Southern Mississippi, USA, Dr. Zhe Qiang and group have found a perfect solution to mitigate global mask pollution. They have recently upcycled waste surgical/face mask into high value carbon fibers – via simple steps including thermal stabilization and pyrolysis – which can fully retain the initial fiberous structure of polypropylene in the mask. The work is published in ‘ACS Omega’ [4].
Before upcyling, the authors have cut the surgical mask to remove the elastic bands, metal nosepiece and inner melt-spun mat, and the outer fabric layers of the mask were used to produce carbon fibers. Their strategy involves an initial sulfonation step by dipping the mask in sulfuric acid at 155 °C, which introduces sulfonic acid groups (SO3H) into the secondary or tertiary carbons in the polypropylene chain (see Scheme 1). With continuous heating, these can undergo desulfonation, which results in unsaturated bonds in the polymer backbone that can react with another polymer chain to form crosslinked structure. The second step involves carbonization process by heating thermally stabilized mask at elevated temperature (800-1400 °C), which removes all functional groups (SO2, H2O, CO, CO2, etc.,) to form porous carbonaceous material.
